Jon et al. (2020) document the geographic and geologic landscapes of Mt. Kumgang (38°38´N, 127°59´E) in the DPR Korea and provide a systematic and comprehensive evaluation of the natural- and geo-heritage significance of the region. We argue that Mt. Kumgang, which has a thousand-year-long history of tourism in Korea (Shin, 2016), should be nominated as a natural World Heritage Site to be shared with all citizens of the world. However, we should like to stress that non-glacial processes can form the landforms described as glacial by Jon et al. (2020); and that it is highly unlikely that Mt. Kumgang was glaciated. We do not want to depreciate the geologic and natural heritage value of Mt. Kumgang and of scientific and geologic contributions of Jon et al. (2020). However, our discussion of the varied interpretations will enhance the importance of the Mt. Kumgang as a critical geologic location and the potential for it to become a World Heritage Site.
In their paper, Jon et al. (2020) assert that a glacier existed on Mt. Kumgang during the last glacial period, probably during the global last glacial maximum (LGM; 26 – 19 ka of Clark et al., 2009 or 28 – 23 ka of Hughes and Gibbard, 2015). Their proposed glacier formed in a cirque, thickened to ~100 m and advanced along the valley, eroding the valley making it U-shaped, to the coast where it produced a moraine. However, the evidence for glaciation presented in Jon et al. (2020) is equivocal. Below, we highlight several reasons why their interpretation of the landscape as one fashioned by for glaciation is not robust:
1. Striations: Jon et al. (2020) suggest that elongate scratches, they call striations, in the granite bedrock surface are glacial in origin. Although striations are among the most common features of glacial erosion (Hambrey, 1994), there can also be produced by non-glacial processes (Hovey, 1909; McLennan, 1971; Eyles, 1993; Osborn et al., 2008), particularly by high-energy water flow creating parallel striations by abrading entrained rocks (Richardson and Carling, 2005; Shaw et al., 2020). Mt. Kumgang is subject to torrent floods during the summer monsoon or typhoon season, where sediment-loaded waters can easily lead to scratched bedrock surfaces reminiscent of glacially striated surfaces. We do recognize that photographs of the striations in their Figure 7C and D have remarkable similarity to glacial striations because of the consistent parallelism, however, as discussed in point 2 these are unlikely to have been produced by a LGM glacier.
2. Survival of LGM glacial striations on the contemporary streambed: Jon et al. (2020) argue that the bedrock surface marks in the contemporary streambed are also striations that are glacial in origin. However, it is unlikely that glacial striations in the active bedrock channel would last for some 20,000 years because fluvial abrasion would quickly erode them away. Contemporary fluvial erosion, of course, could produce glacial striations-like forms, as in mentioned in point 1. In particular, the minimum erosion (incision) rate in this region should be > 50 mm/ka, based on cosmogenic 10Be catchment-wide denudation rate measured ~ 50 km south on the Eastern Korea Peninsula (Kim et al., 2016). Considering the monsoon climate and the prevalence of typhoons, the stream bedrock surface’s erosion rate would be much greater than 50 mm/ka. The published erosion rate of an active stream granite bed measured ~ 150 km south yields even higher rates of 200 – 500 mm/ka (Lee et al., 2012), thus potentially removing > 20 m of the stream bed since the LGM. The likelihood of any glacial striations surviving in the contemporary streambed since the LGM is negligible.
3. The age of glacier: Jon et al. (2020) suggest the glacial age of ca. 28 ka based on the fission track dating. As far as we know, however, there has been no study that tried to determine the glacial age by fission track dating, and the glacial ages they reported are hard to accept for two reasons. First, they argue that the glacial age can be determined by fission track dating because of the total annealing of tracks due to the frictional heat and shock power. According to the experiment by Schmidt et al. (2014), pressure has no significant effect on fission track annealing, at least at the upper crustal level under which thermal annealing of track occurs in apatite. If so, the only factor affecting track annealing is frictional heat. However, it is not possible to raise the temperature high enough to cause track annealing due to glacial friction. Moreover, considering the shot heating time, the temperature required for track annealing should be much higher. Secondly, it is difficult to measure the age of less than 500 ky using the apatite fission track dating because of the low uranium contents of apatite (10 - 100 ppm). Their experimental results show the extremely low spontaneous track density (ρs: 1.09 ~ 1.21 × 103 cm-2). In such cases, the error is very large and dating results are unreliable. In addition, Jon et al. (2020) did not provide essential information such as Ns (number of spontaneous tracks), Ni (number of induced tracks), dosimeter glass, and calibration constant (ρs-value), so the reliability of their results is very low.
4. Cirque: A bowl-shaped depression high up Mt. Kumgang is interpreted by Jon et al. (2020) as a cirque. However, non-glacier processes can produce such landforms, and several similar forms are present in the area that are not considered glacial in origin (Fig. 5 in Jon et al., 2020). Moreover, these depressions are not typically cirque-shaped, as compared with those on glaciated mountains elsewhere in the world (Evans and Cox, 1974; Evans, 2021), including at Mt. Kwanmobong (41°30´N, 129°12´E; Rhee et al., 2015) and at Mt. Baekdu (42°00´N, 128°03´E; Zhang et al., 2009). Also, there is insufficient space (flat hollow), and the slope is too steep (> 40° in the valley heads) for snow to accumulate and for a glacier to develop. The “cirques” described by Jon et al. (2020) are more likely to be normal valley heads as are found in many high and steep mountains. Alternatively, they could be nivation hollows.
5. U-shaped valleys: Jon et al. (2020) described the valley as U-shaped and that this is indicative of glaciation. However, the valley cross-profiles are more V-shaped with low valley floor width to valley height ratios (Figure 1; McGregor et al., 2000; Seong et al., 2008). Also, there are no trimlines or glacier striations up to 100 m above the valley floor in the valley, the height corresponding to the thickness of their proposed glacier. Hence the shape of the valley does not provide evidence to support the existence of a 100-m-thick glacier.
6. Hanging falls and step-pools: Jon et al. (2020) argued that falls and pools of various sizes are characteristic of a glacial eroded landscape. However, fluvial steps and pools can form on granite without the need for glacial processes (Carling et al., 2005), especially when the bedrock has a well-developed joint system. In this case, most of the hanging falls and step-pools suggested to be glacial are coincident with the locations of the joint system trending northwest, which can help produce such features by differential fluvial erosion (Figure 2). Various sizes (1 - 10s m2) of step-pool sequences developed by active headward erosion and cascades are common features of granite mountains in south Korea (Figure 3).
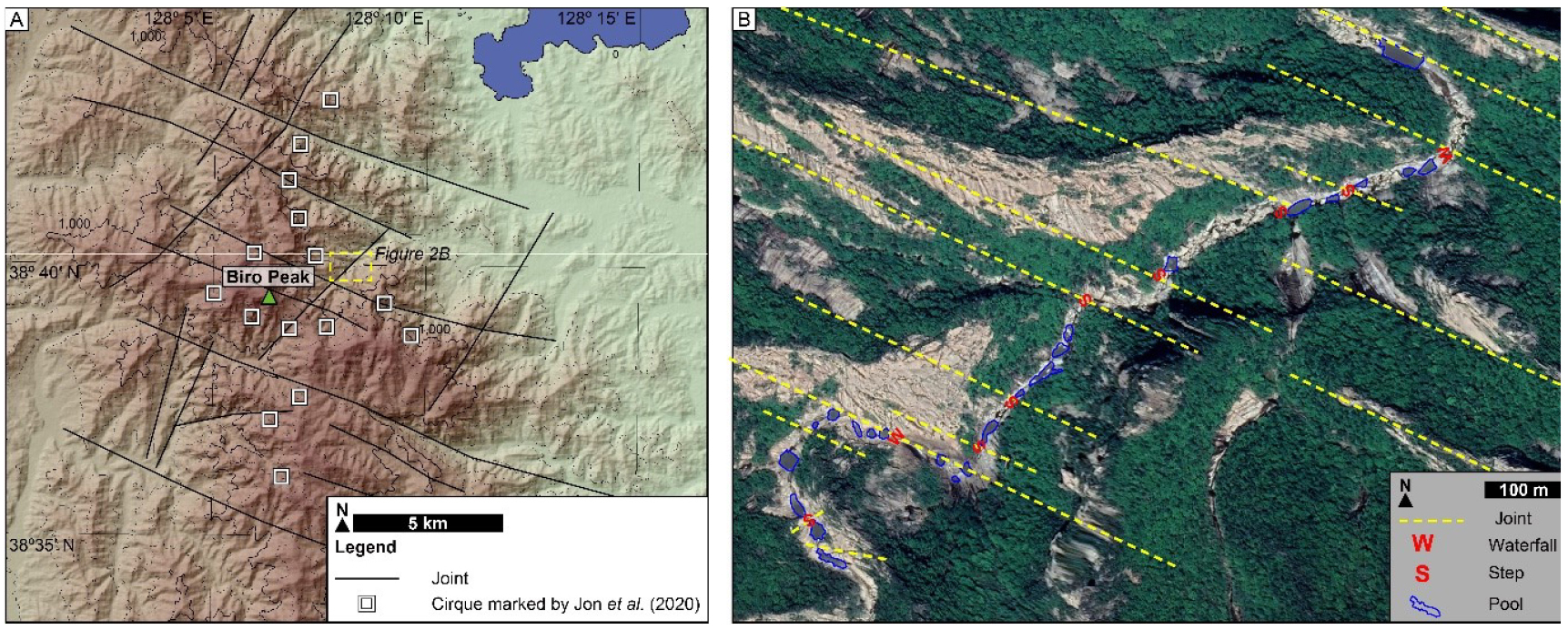
Figure 2
Distribution of the NW-trending master joint system in Mt. Kumgang. Locations of (A) the proposed cirques of Jon et al. (2020) and (B) Waterfalls, step, and pools along the Guyongyeon River. White boxes are the cirques proposed by Jon et al. (2020).
7. Moraine: Jon et al. (2020) suggested that a ridge-shaped landform composed of diamict near the coast is a moraine. However, diamicts and such landforms have been misinterpreted in many regions as glacial in origin when many have been shown to be non-glacial in origin (Derbyshire, 1983; Hewitt, 1999; Osborn et al., 2008 and references therein). The authors simply assume the diamict to be glacial by citing previous works on moraine deposits at different locations with similar latitudes, but they provide no supporting sedimentological criteria (Kim et al., 1999). Moreover, to produce a moraine at the coast would need an ELA change of ~ 900 m, which is highly unlikely at this latitude of ~ 38°N. A more straightforward explanation is that the diamict is a debris flow deposit.
8. Equilibrium-line altitude (ELA): The high peaks of Mt. Kumgang rise to ~ 1600 m above sea level (asl), and if any glacier existed during the last glacial period, the former ELA would need to have been at an elevation of circa 1400 m asl to allow enough accumulation area space for the glacier to develop. The ELAs reconstructed from the cirques in Korea and Japan at similar latitudes for the LGM are ~ 2200 m asl at Mt. Kwanmobong, 41°N (Rhee et al., 2015) and ~ 2500 m asl at the northern Japanese Alps, 37°N (Ono et al., 2005). This observation indicates that the ELA would have been at least 800 m lower than other areas of similar latitude in East Asia based on the published compilation data for the Northern Hemisphere (Mitchell and Humphries, 2015). Although there can be variation in ELA due to local topographic and climatic control, the ELA for the LGM should be > 2200 m. Defining a ~ 1400 m ELA of Mt. Kumgang makes little sense with respect to regional glaciology and climatology.
9. Geographic context: To date, only two locations are confirmed by glacial geologists/geomorphologists to have glaciers in the DPR Korea during the last glacial period, Mt. Baekdu/Mt. Changbai on the border of China and North Korea (Zhang et al., 2009; Lee et al., 2012) and Mt. Gwanmobong in North Korea (Sasa and Tanaka, 1938; Rhee et al., 2015). Although others, such as Kim et al. (1999) argue, that there are > 70 places that preserve glacier-related landforms across the DPR Korea, it is highly unlikely that Mt. Kumgang was glaciated.
Although we argue that the landforms on Mt. Kumgang are not glacial in contrast to Jon et al. (2020), the massif has a large variety of rocky landforms ranging from small pools to grand peaks and valleys. These impressive landforms have helped attract local tourism for over a thousand years, making this a place an area of outstanding natural beauty and one worthy of world recognition.